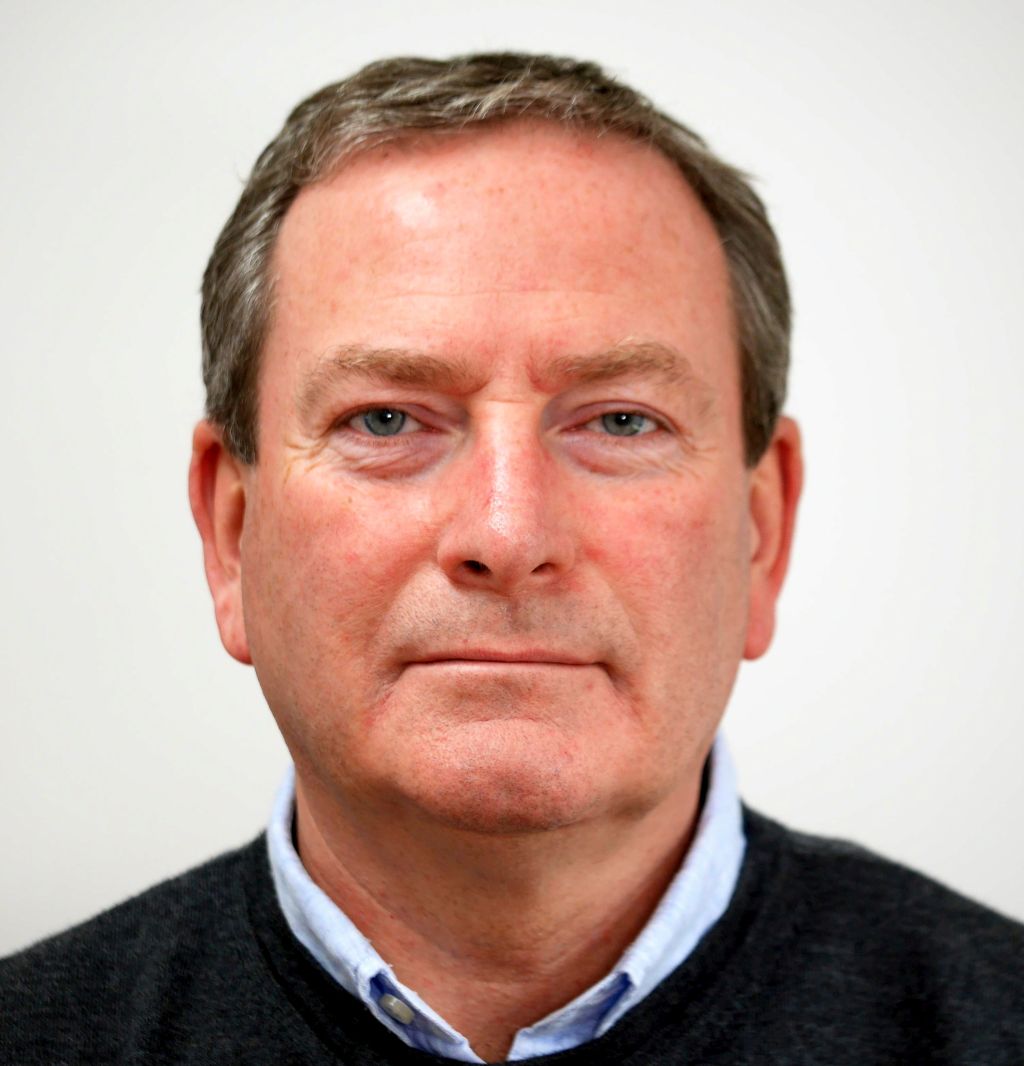
David Boettcher - Professional Engineer
Eur Ing D B Boettcher BSc(Hons) CEng MIET
Providing innovative solutions to engineering and business problems opportunities.
Nuclear Power
I joined the nuclear power industry in 1975, working for what began as the Atomic Power Division (APD) of English Electric. The names of companies and consortia in the British nuclear power industry changed with baffling frequency and I won't repeat them all here.
I initially worked in the Research and Development (R&D) laboratories, and then after graduating in the Reactor Engineering Department (RED) mainly sorting out construction and commissioning problems in the reactors and fuel routes of Advanced Gas Cooled Reactors (AGRs), and life extensions of the Magnox reactors. My first nine years were spent working on the nuclear power stations Hartlepool, Heysham I, Dungeness B, Hinkley Point A and Tokai Mura.
In 1984 I transferred to the Systems and Safety department of the Sizewell B project, working on the Public Inquiry and safety case for the Control and Instrumentation (C&I) and electrical systems, including the Reactor Control System (RCS), Reactor Protection Systems (PPS and SPS), the Station Control and Data Acquisition systems (SCADA), the Main Control Room (MCR) and Auxiliary Shutdown Room (ASR) and the safety related electrical systems and back-up diesel generators. I also worked on proposals for replicas of Sizewell B to be built at Hinkley Point C and in Taiwan as the Lungmen (Dragon Gate) nuclear power plant.
During my time in the nuclear power industry I was exposed to many aspects of the construction of Nuclear Power Stations (NPS), their engineering, nuclear safety, and economics. Here I record a few thoughts about these aspects.
The Economics of Nuclear Power
Magnox nuclear power stations produced electricity that was considerably more expensive than electricity produced by coal fired power stations. However, in the 1960s the UK government decided to order construction of a series of Magnox power stations to provide an alternative source of electricity to that generated by coal fired power stations, and thus weaken the power of the miner's unions. It was hoped that the Magnox design could be developed so that the electricity they produced would be cheaper, but this development programme ran into a brick wall when it was found that the higher temperatures resulted in far higher rates of corrosion of the mild steel components than had been envisaged. It turned out that instead of increasing linearly with temperature, above a certain temperature around 400°C the rate of corrosion was much greater than below. This was termed "breakaway corrosion" and the later Magnox stations had to be "downrated" (run at lower than designed power output) because of this.
The Advanced Gas Cooled Reactor (AGR) nuclear power stations were designed to overcome this problem, using stainless steel to allow higher temperatures which increased their thermal efficiency - but their designers still needed to call on the "plutonium credit" to prove that they were economic. However, the AGRs were so complicated that massive delays occurred during construction, pushing up costs.
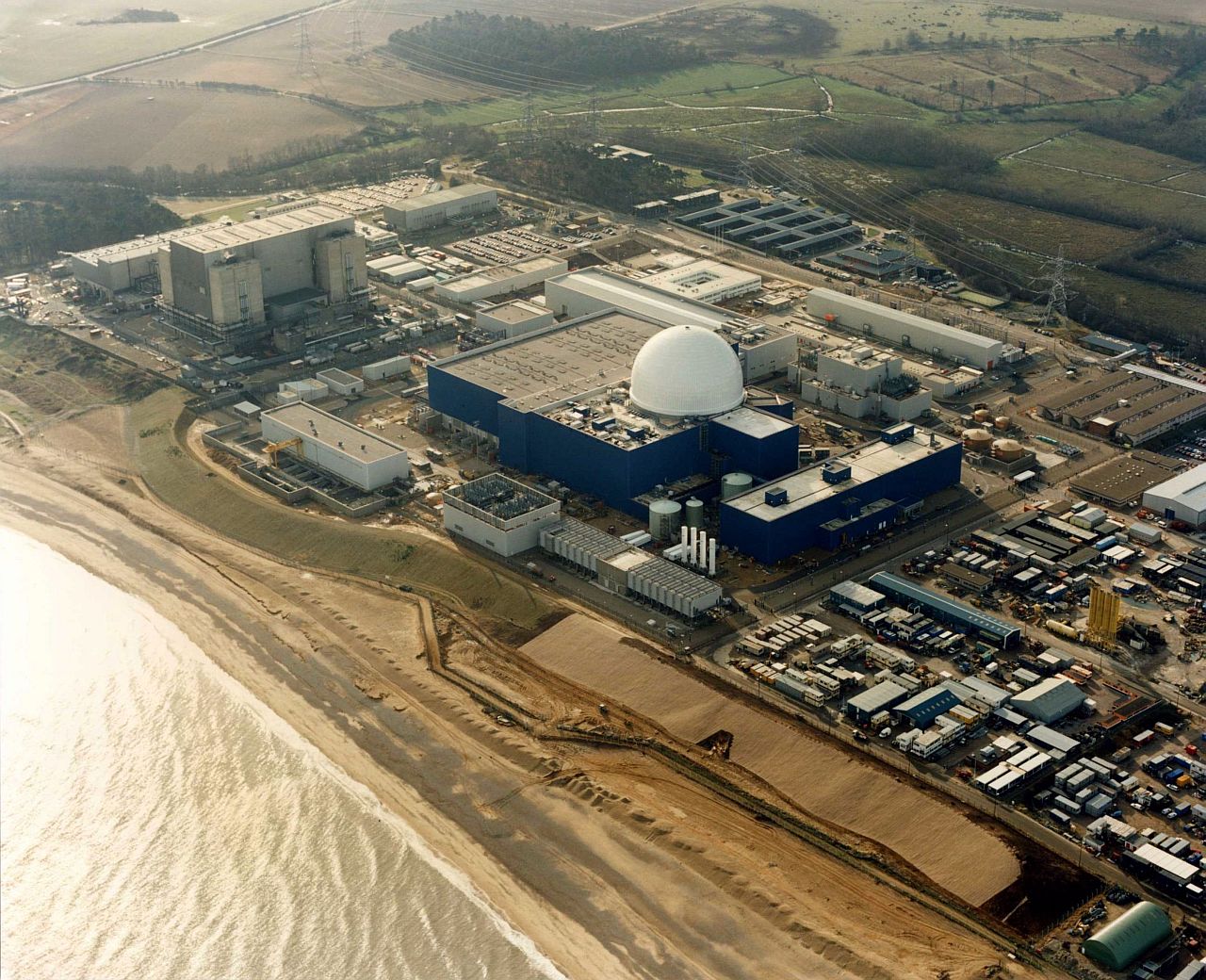
Sizewell B at a late stage of construction, with Sizewell A to the upper left of the picture: click to enlarge, ← to return.
The Pressurised Water Reactor (PWR) design was then tried, and the construction of Sizewell B, on which I worked for 10 years, went to time and budget. But the out turn cost, the final cost of construction, was high - £2.030bn - yes, that's over two billion pounds, which back in 1994 was a lot of money. Nevertheless, under Margaret Thatcher's leadership, the Conservative Government planned to instruct the Central Electricity Generating Board (CEGB - a nationalised company and therefore under Government control) to construct 10 more plants like Sizewell B. We began to plan for further PWR power stations at Hinkley Point, Druridge Bay, Wylfa on Anglesey and a second PWR at Sizewell.
The picture to the right shows Sizewell B, with the Magnox Sizewell A in the upper left corner. The blue building in the middle with the white dome on top is the Sizewell B "nuclear island", an earthquake-proof structure which contains the reactor and all the essential bits and bobs that look after it; the white dome covers the reactor building itself. Behind and to the left adjoining the nuclear island is another blue building, this one with a flat roof; this is the turbine house, housing two nominal 660MW turbo-alternator sets. In front to the right of the nuclear island is the radwaste building which process radioactive waste and the spent fuel. Surrounding these principal buildings are other buildings and structures containing the sea water intake pumps, standby diesel generators, the reserve ultimate heat sink, and the switchgear that connects the power station to the outgoing power lines. As you can see, it is a massive plant for the its electricity output of around 1,170MWe.
I worked on the Public Inquiry for Hinkley Point C, which examined the proposal to build the first replica of Sizewell B at Hinkley Point in Somerset. We could make the economic case that the electricity produced would at a price competitive with that produced by a coal fired power station, IF we used a required 5% rate of return on investment (standard for Government contracts at the time) AND assumed that the coal would cost £135 per ton at the pithead of a Welsh colliery.
In 1989 the Central Electricity Generating Board was broken up and the parts "Privatised" - sold to private investors as Publicly Listed Companies ("PLCs") which were therefore no longer under Government control. After being involved in the project management of Sizewell B and the Public Inquiry for Hinkley Point C, I well understood the costs and financial risks involved, and it was clear to me that no private company would (or even could) invest billions of pounds into a project that could take up to 10 years or more to begin generating power for a 5% return on the capital they had invested. Especially if they factored in the "project risk" - the risk that at any moment a disaster at a nuclear power plant could mean that the station would never obtain a licence to operate.
At the time, coal was available much cheaper than £135 a ton: it could be had from from Australia landed at the dockside in the UK for £35 per ton - and gas was even cheaper: gas fired power stations could be built in three years for a tiny fraction of the cost of a nuclear power station, and produce a rate of return on investment above 20%. The economics of nuclear power clearly didn't stack up and it was obvious that no private company would build a new nuclear power station, so at the end of my work on Sizewell B, I left the industry.
Then Global Warming was discovered, and nuclear was an obvious way to cut carbon emissions . . . . but there still haven't been any more nuclear power stations built in the UK - and there wouldn't be until be the government guarantees decent profit incentives and "project risk" safeguards to the constructors, that the consumer will end up paying for one way or another for decades to come. Even then, it is unlikely to be private companies that can afford to take on the huge investments in exchange for the very long payback periods involved. The only entities with that sort of finance, long term planning and requirement for steady predictable streams of income for decades to come are governments. Unless the British Government funds the construction of the "next generation" of nuclear power stations, either directly with British tax payer's money or indirectly through a hypothecated levy on consumer's bills, the French or Chinese governments will put up the capital in exchange for a guaranteed (high) price for the electricity generated, which will increase home energy bills and British electricity consumers will end up paying the state pensions of French or Chinese pensioners long into the future.
In February 2013, Centrica pulled out of the project to build a new nuclear power station at Hinkley Point C, saying that "the anticipated project costs in new nuclear have increased and the construction timetable has extended by a number of years. These factors, in particular the lengthening time frame for a return on the capital invested in a project of this scale, have led us to conclude that participation is not right for Centrica and our shareholders." Surprise? No - well, not to me.
In 2014 the price for Hinkley Point C plant estimated that its two reactors will cost £8bn each (although construction hasn't started yet and the cost of the two reactors of this type that are being built, at Flamanville and Olkiluoto, are running late and over budget) and that the consortium led by EdF have been guaranteed a minimum price of £92.50 for every megawatt hour (MWh). The price is also index-linked to inflation, meaning EDF can expect about £121 per MWh by 2023. (These figures are probably a bit out of date now, but in the nuclear construction industry costs never go down, so I can guess how they will go.) When I was looking at building a replica of Sizewell B at Hinkley Point, we were talking about £28 per MWh (2.8 pence per kilowatt hour) wholesale as being economic. How things have changed.
Oh, and don't forget, we still need to figure out what to do with the spent fuel and nuclear waste and figure the cost of that into the equation. A nuclear power station turns a large amount of innocuous material into a large amount of very radioactive material during its operating lifetime. I'm not saying that building more nuclear power stations is wrong mind: global warming turns conventional thinking about the economics of nuclear power on its head. But we do need to go into it knowing all the facts and costs over the long term. I don't think this has been properly considered for the proposed new nuclear power plants that are now being built.
The Safety of Nuclear Power
A new nuclear fuel rod that has never been near a reactor is quite safe to handle without any particular safety precautions or shielding - I have stood next to many AGR fuel elements consisting of a graphite sleeve and 36 enriched uranium fuel rods. However, once the fuel is "burned" (irradiated) in a reactor, highly radioactive fission products accumulate in the fuel rod. To come close to such a fuel rod would be quickly fatal. However, so long as the fission products remain in the fuel rod, and the fuel rod is kept behind an effective shield, there is no significant risk of damage to human health, although there will always be a small amount of radioactivity released to the environment from nuclear power stations and there is statistically a small risk, which needs to be outweighed in some way by the benefit of the power produced. At one time this would have been said to be the low cost of the electricity, but in reality nuclear generated electricity has never been cheap. But today, the benefits of low carbon generation electricity can reduce global warming.
If the fission products escape from the fuel rods into the environment, there is a significant risk to human health. When the fuel rods are in the reactor and the reactor is critical, the fission reaction in the fuel rods produces a lot of heat, but this is safely removed by the reactor cooling system and used to generate steam, which powers the turbine generator that generates electricity. If there is a problem with the reactor cooling system which impairs its ability to remove the fission reaction heat from the fuel, the reactor is automatically shut down and the fission reaction stopped. However, this does not bring the amount of heat produced by the fuel to zero. The fission products in the fuel decay even after the fission reaction has stopped, producing "decay heat". Although this is much less than the heat produced by the fission reaction, it must still be removed to prevent the fuel rods overheating and melting, releasing the fission products.
Immediately after the reactor has been shut down, a fuel rod produces about the same amount of heat per unit length, i.e. per foot or per metre, as a common domestic kettle element. So long as the fuel remains covered by water, even at only atmospheric pressure, the water will adequately carry away the decay heat. However, if the water boils away and the fuel is uncovered, then it will melt and release fission products. This is what happened to the reactors and spent fuel pools at Fukushima Daiichi in 2011, and to the reactor at Three Mile Island in 1979.
So in principle, ensuring nuclear safety just means that you need to keep the fuel covered with water. But of course, in practice there is a lot more to it than that. However, if this simple principle had been able to be followed at Three Mile Island and Fukushima Daiichi, you would almost certainly never have heard these names in the news. The problem at Three Mile Island was one of ergonomics and training, the power station operators didn't understand what was happening in the reactor and switched off the safety systems which were keeping the fuel covered with water. You can read more about this on my ergonomics page.
The problem at Fukushima Daiichi was that the safety systems that would have kept the fuel in the reactors and spent fuel pools covered with water were not designed to withstand a magnitude 9 earthquake, although it seems that they did, and the ensuing 14 metre high tsunami, which they didn't because it flooded essential electrical switchgear. At the time the Fukushima Daiichi plant was designed, in the 1960s or 1970s, the American Code of Federal Regulations 10CFR50 decreed that nuclear plants should be designed to withstand "once in 100 years" magnitude events, and most nuclear power plant designers followed this rule. Unfortunately the earthquake and tsunami were bigger than the events that this simple rule predicted and the plant's safety systems were inundated, causing their failure. This is what designers call a "beyond design basis event", an innocuous phrase meaning "we didn't design it to withstand that".
Spent fuel rods that are not adequately cooled heat up, and the zircalloy (zirconium alloy) cladding (casing) around the contained uranium fuel reacts with steam given off by the boiling water. The cladding oxidises by taking oxygen from the steam, in the process releasing hydrogen gas. This hydrogen gas can mix with air to form an explosive mixture. It was hydrogen-air explosions that blew apart the buildings on top of the reactors at Fukushima Daiichi in the early days of the accidents and that told me that fuel was melting, despite news reports to the contrary being released. The situation at Fukishima is something that the plant was not designed to cope with and, like Chernobyl, requires improvised clean up methods that will be very expensive and long term.
Copyright © David Boettcher, 2006 - 2025 all rights reserved. Please feel free to contact me via the Contact me page.
This page updated November 2019. W3CMVS.